Research in the Hovel lab
Investigations in marine conservation ecologyOverview
Research in SDSU’s Marine Conservation Ecology lab focuses on the interaction of invertebrate and vertebrate animals and their benthic habitats. Our projects take place in seagrass beds, nearshore rocky reefs, estuarine environments, kelp forests, and coral reefs. We have a dual focus of exploring ecological concepts and addressing real-world problems that affect species and habitats in coastal ecosystems. We use a combination of field experiments, lab experiments, and mathematical modeling to address our ecological questions.
Our research is funded by the National Science Foundation (NSF), California Sea Grant, the California Ocean Science Trust, the National Marine Fisheries Service (NMFS), and local organizations like the Unified Port of San Diego. Students in the lab have received funding from a variety of national, state, and local institutions, notably CSU COAST‘s Kenneth H. Coale Graduate Scholar Award, NSF Graduate Research Fellowships, California Sea Grant, and internal SDSU grant programs such as the ARCS program in the College of Sciences (please go to our People page for specific examples).
SEAGRASS ECOLOGY
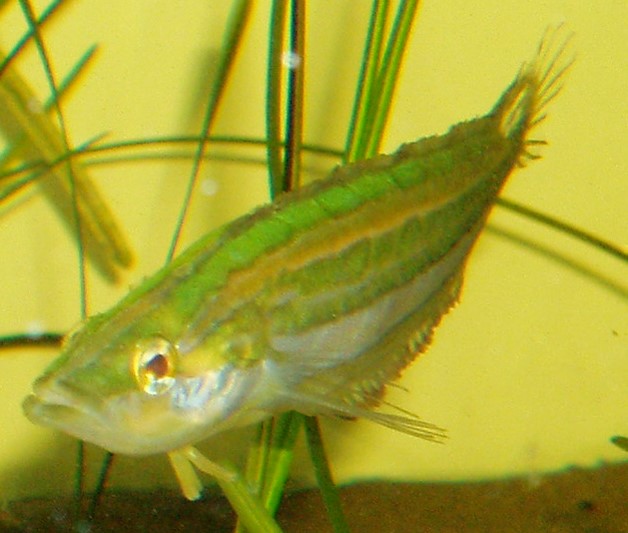
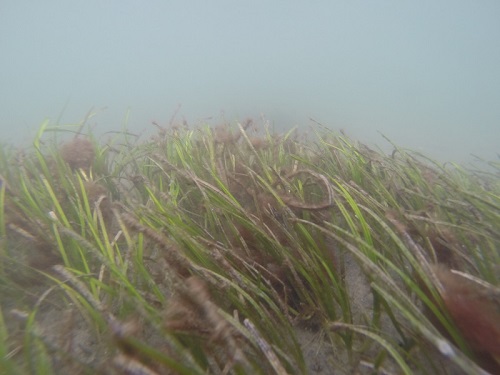
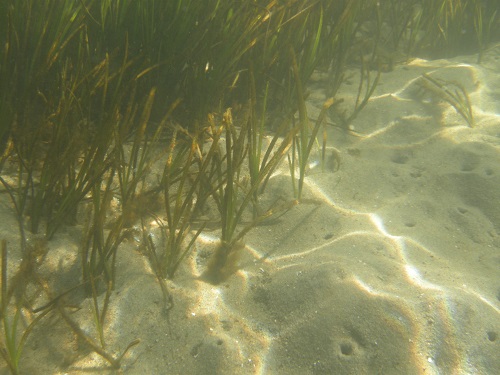
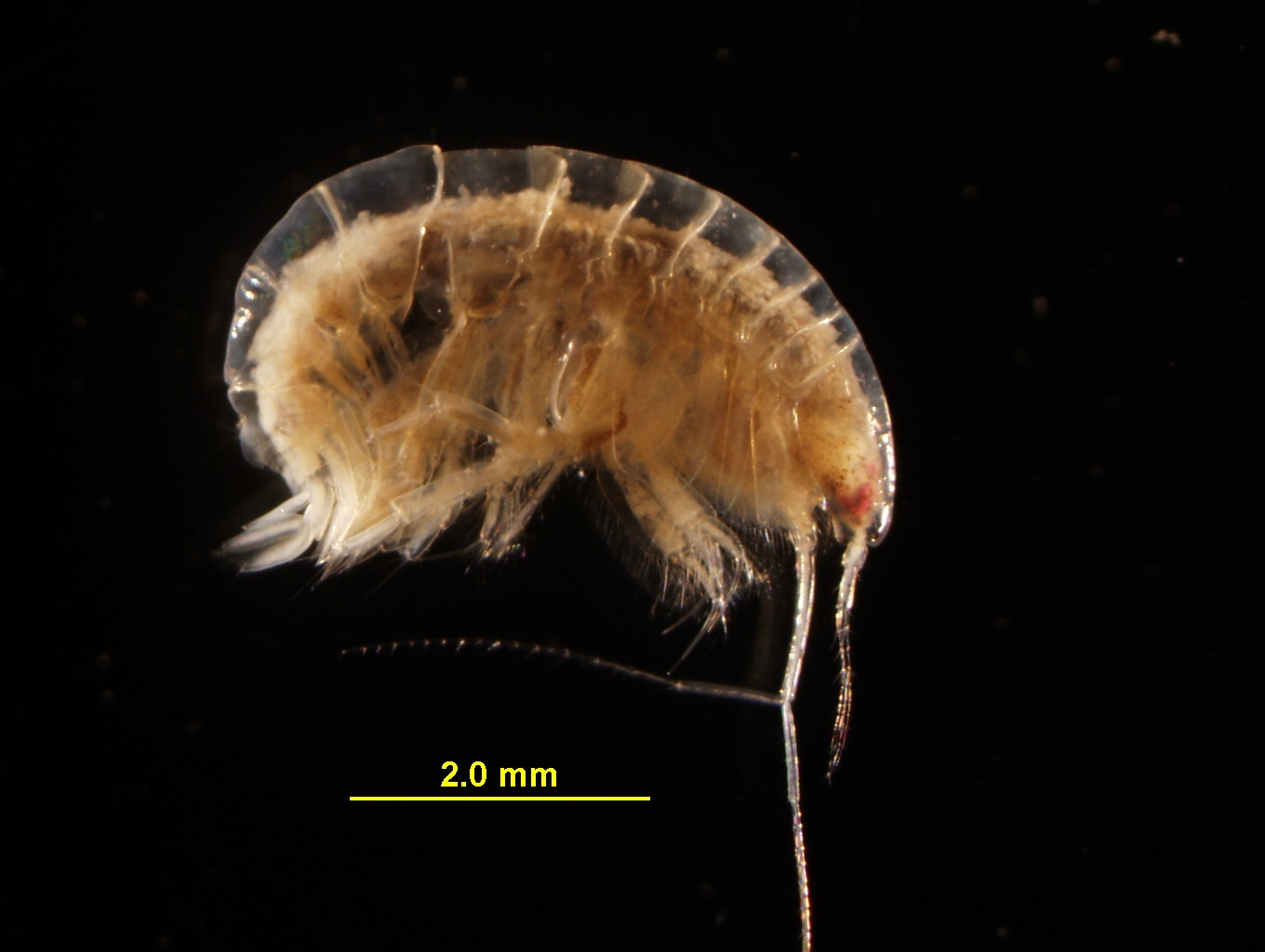
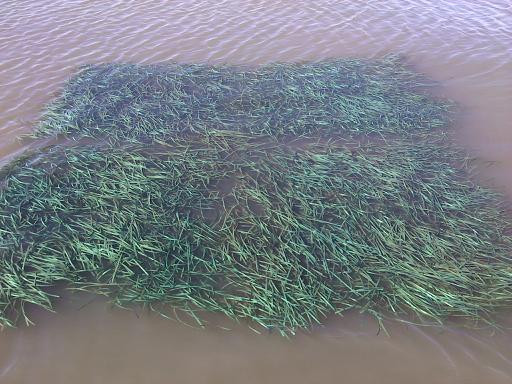
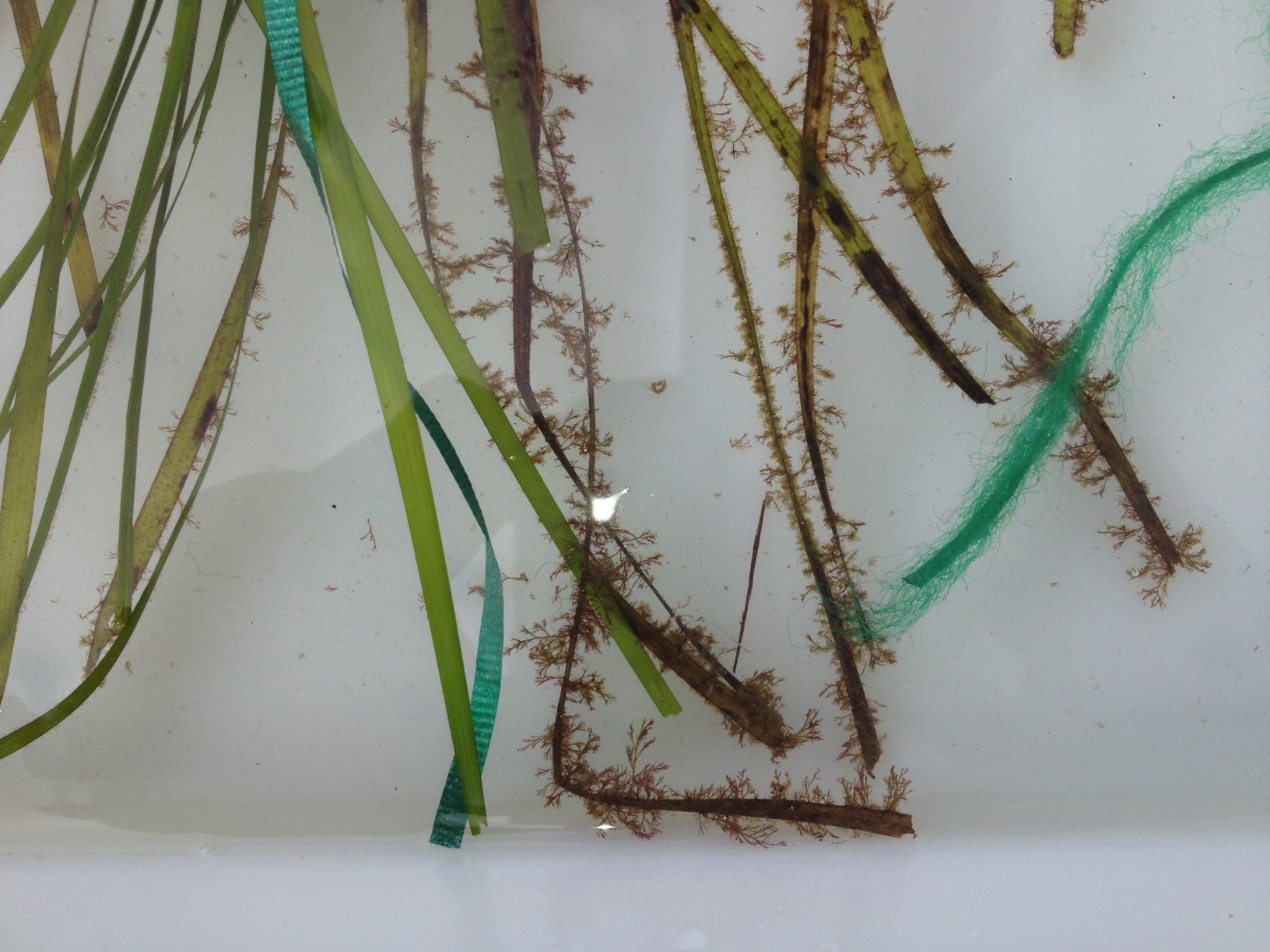
Seagrass habitats are some of the most valuable habitats in the oceans. This is true both ecologically and economically. Eelgrass, one of about 60 species of seagrasses, is one of the most widespread of all marine macrophytes, occuring on all continents except Antarctica. Eelgrass provides a variety of ecosystem services, including providing habitat to fishery species, sequestering carbon, buffering shorelines from erosion, and reducing aquatic contaminant loads.
The world’s seagrass ecosystems are declining, with nearly 30% of global seagrass habitat being lost over the last several decades. This makes understanding the ecology of these systems all the more important.
Investigations in eelgrass ecology make up one of the major research themes in our lab. We ask several fundamental questions regarding eelgrass habitat, such as:
- how does eelgrass habitat structure influence predator-prey interactions and animal biodiversity?
- what is the role of “top-down” processes, such as predation and herbivory, in sustaining eelgrass habitats?
- what is the relationship between eelgrass habitat structure, faunal community structure, and key ecosystem functions?
Eelgrass habitat structure and predator-prey interactions
I am particularly interested in the effects of habitat structure on mesopredators. Mesopredators are (often) juvenile fishes or invertebrates that inhabit eelgrass for refuge from larger predators. But these vulnerable juveniles also are hunters of smaller prey. Thus, they constantly face decisions about how to best avoid being detected by larger organisms and how to efficiently forage on smaller organisms. Habitat structure may strongly influence both of these functions. The density and biomass of eelgrass shoots within patches (often referred to as structural complexity) influences how easily mesopredators are detected, how well they move, and how easily they can detect prey. Eelgrass habitat is often patchy, and proximity to patch edges may influence how often mesopredators encounter larger predators that tend to patrol these edges. Finally, at seascape scales, patchiness and connectivity of the seascape influences their ability to move throughout an area, how they select patches for foraging and refuge, and their vulnerability as they do so.
Many of my graduate and undergraduate students have explored these issues for local San Diego mesopredators such as the juvenile giant kelpfish. Recent projects have addressed issues like:
- how do the effects of habitat structure on fish foraging and survival vary through ontogeny?
- what are the effects of climate change, such as acidification and ocean warming, on predation and herbivory?
- how does structural complexity affect habitat selection, and are these decisions modified by food availability and predation risk?
- what are the relative influences of structural complexity and prey density on foraging efficiency?
- how does eelgrass patchiness influence habitat use, predation risk, and growth?
Relevant publications:
Hovel, K.A., A.M. Warneke, S.P. Virtue-Hilborn, and A.E. Sanchez. 2015. Mesopredator foraging success in eelgrass (Zostera marina L.): relative effects of epiphytes, shoot density, and prey abundance. Journal of Experimental Marine Biology and Ecology 474: 142-147.
Tait, K.J. and K.A. Hovel. 2012. Do predation risk and food availability modify prey and mesopredator microhabitat selection in eelgrass (Zostera marina) habitat? Journal of Experimental Marine Biology and Ecology 426: 60-67.
Lannin, R. and K.A. Hovel. 2011. Variable prey density modifies the effects of seagrass habitat structure on predator-prey interactions. Marine Ecology Progress Series 442: 59-70.
Hovel, K.A. and H. M. Regan. 2007. Using an individual-based model to examine the roles of habitat fragmentation and behavior on predator-prey relationships in seagrass landscapes. Landscape Ecology DOI 10.1007/s10980-007-9148-9.
Eelgrass habitat structure and faunal community structure
Another focus of our lab’s seagrass research is on how habitat structure influences the abundance and diversity of epifaunal organisms (the organisms that live among and on eelgrass blades). We address the influence of structural complexity on community structure, as well as the influence of features at the seascape scale, such as patch connectivity, habitat fragmentation, and proximity to edges. And many of our projects focus on how structure at these two scales interact to structure communities, a concept that is greatly understudied in the sea as well as in terrestrial habitats.
Relevant publications:
Mizerek, T., H.M. Regan, and K.A. Hovel. 2011. Habitat loss and fragmentation levels determine optimal management of invertebrates in seagrass habitats. Marine Ecology Progress Series 427: 247-257.
Moore, E.C. and K.A. Hovel. 2010. Relative influence of habitat complexity and proximity to patch edges on seagrass epifaunal communities. Oikos 119: 1299-1311.
Sirota, L. and K.A. Hovel. 2006. Eelgrass (Zostera marina) structural complexity: relative effects of shoot length, shoot density, and surface area on epifaunal community composition in San Diego Bay, California, USA. Marine Ecology Progress Series 326: 115-131.
Reed, B.J. and K.A. Hovel. 2006. Seagrass habitat disturbance: how loss and fragmentation of eelgrass (Zostera marina) influences epifaunal abundance and diversity in San Diego Bay, California, USA. Marine Ecology Progress Series 326: 133-143.
Healey, D. and K.A. Hovel. 2004. Seagrass patchiness influences epifaunal abundance and diversity in San Diego Bay, USA. Journal of Experimental Marine Biology and Ecology 313: 155-174.
Ecosystem function in eelgrass habitats
A recent focus for our lab has been the investigation of links between habitat structure, faunal community structure, and important ecosystem functions. Ecosystem functions include the influence of herbivory on eelgrass growth, the production of epifaunal consumers (important links in seagrass food webs), the storage of carbon, and the cycling of nutrients.
In 2011, our lab joined the Zostera Experimental Network, led by Dr. Emmett Duffy of the Smithsonian Institution in Washington, D.C. This network is a collaboration of scientists from across the northern hemisphere that study linkages between eelgrass habitat, biodiversity, and ecosystem function. The first major focus of ZEN was to investigate the relative effects of top-down (herbivory) and bottom-up (nutrients) effects on eelgrass growth and persistence. Specifically, we tested the hypothesis that epifaunal consumers in eelgrass habitat help maintain productive eelgrass beds by grazing on competing epiphytic algae. A major result from this study was that globally, sites with high biodiversity of epifaunal consumers had the largest top-down effects on epiphytic algae. This result confirmed the importance of biodiversity, which had been shown to have many positive effects on important ecosystem functions in a variety of laboratory experiments and singular field experiments.
ZEN now has expanded to focus on several linkages between epifauna, eelgrass structure, and ecosystem function. A recently funded proposal authored by Dr. Emmett Duffy, Dr. Jay Stachowicz, and myself has funded the last three years of investigation into these issues. Some recent experiments have examined:
- how eelgrass structural complexity and proximity to patch edges jointly influence mortality risk for epifauna;
- how eelgrass structural complexity and predators influence epifaunal grazing rates on epiphytic algae;
- global patterns of mortality risk for epifauna, and the factors that influence global trends in mortality risk;
- linkages between eelgrass genetic diversity, structural complexity, and epifaunal abundance and diversity;
- linkages between epifaunal functional traits and ecosystem function.
Relevant publications:
Duffy, J.E., P.L. Reynolds, C. Boström, J.A. Coyer, M. Cusson, S. Donadi, J.G. Douglass, J. Eklöf, A. Engelen, B.K. Eriksson, S. Fredriksen, L. Gamfeldt, C. Gustafsson, G. Hoarau, M. Hori, K.A. Hovel, K. Iken, J.S. Lefcheck, P.O. Moksnes, M. Nakaoka, M.I. O’Connor, J.L. Olsen, J.P. Richardson, J.L. Ruesink, E.E. Sotka, J. Thormar, M.A. Whalen, and J.J. Stachowicz. 2015. Biodiversity mediates top-down control in eelgrass ecosystems: A global comparative-experimental approach. Ecology Letters DOI 10.1111/ele.12448.
Reynolds, P.L., J.J. Stachowicz, K.A. Hovel, C. Boström, K. Boyer, M. Cusson, J.S. Eklöf, F.G. Engel, A.H. Engelen, B.K. Eriksson, J. Fodrie, J.N. Griffin, C. Hereu, M. Hori, T. Hanley, M. Ivanov, P. Jorgensen, C. Kruschel, K.S. Lee, K. McGlathery, P.O. Moksnes, M. Nakaoka, F.T. Nash, M.I. O’Connor, N. O’Connor, R.J. Orth, F. Rossi, J. Ruesink, E. Sotka, R.K.F. Unsworth, M.A. Whalen, and J. E. Duffy. 2017. Latitude, temperature and habitat complexity predict predation pressure in eelgrass across the Northern Hemisphere. Ecology 99: 29-35.
Ruesink,J.L., P.L. Reynolds, C. Boström, M. Cusson, J. Douglass, J. Eklöf, A. H. Engelen, M. Hori, K. Hovel, K. Iken, P.O. Moksnes, M. Nakaoka, M.I. O’Connor, J.L. Olsen, E.E. Sotka, J.J. Stachowicz, *M.A. Whalen, J.E. Duffy. 2017. Global variation in form-function relationships in a marine foundation species and experimental tests of responses to multiple stressors. Oikos. DOI 10.1111/oik.04270
SPINY LOBSTER ECOLOGY
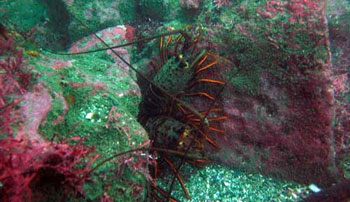
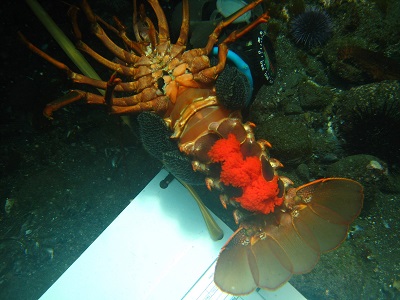
A female spiny lobster holding fertilized eggs on her abdomen. Lobsters may release over 500,000 larvae at a time.
a
The California spiny lobster supports a regionally important fishery in California and Baja California, Mexico. Spiny lobsters are captured commercially in traps, and recreationally by hoop net or by hand while free diving or SCUBA diving. Lobsters also play key roles in kelp forest ecosystems: as an upper-level consumer, lobsters may help control populations of sea urchins, the dominant herbivore in kelp forests. Sea urchins undergo periodic and localized population booms and may form “urchin barrens” which are areas completely devoid of kelp and other algae. Because these algae support diverse communities of invertebrates and fishes, uncovering the processes that dictate the degree of control that lobsters and other predators have on urchins is important, and had been one focal point of our research.
Lobster behavior also has been a focus of our research. In a set of experiments spanning three years, we tracked dozens of spiny lobsters using acoustic tags and hydrophones. Acoustic “pingers” were attached to lobsters, and these animals were tracked for periods of weeks to months. This helped us identify home ranges, habitats used by lobsters as they forage at night, and the distances moved (and movement patterns used) by lobsters as they hunt for prey.
During the daytime, lobsters inhabit shelters made from rocky crevices, stacked boulders, cobble, and hollowed out kelp holdfasts. They can be found singly in shelters, or in groups of up to several dozen lobsters. Some of our recent experiments have focused on the factors that may dictate how lobsters select shelters, and the benefits that they may get from inhabiting particular shelters. For instance, a recent master’s project by Amalia Harrington revealed that juvenile California spiny lobsters select shelters occupied by larger conspecifics, and that this reduces their chances of being consumed by predators.
One of our most recent projects involved quantifying spiny lobster abundance, behavior, and habitat use in a set of southern California marine protected areas (MPAs). California’s Marine Life Protection Act mandated the creation of a network of MPAs throughout state coastal waters. We worked with commercial fishermen and state resource managers to tag and recapture lobsters, in order to quantify population size and movements into and out of MPAs. The results of this work were submitted to the California Ocean Science Trust, the organization that is spearheading MPA baseline monitoring in intertidal and subtidal habitats throughout California.
In 2019, we received funding from California’s Prop 84 program to conduct research on lobster reproductive value throughout the species’ range. The rationale for this project was to update the metrics used in fishery management models that are an important component of the spiny lobster fishery management plan. For example, the spawning potential ratio (SPR) estimates the loss of reproductive capacity of the population due to fishing, which removes reproductively viable lobsters. Accurate estimates of SPR are not possible without information about lobster egg production, size at maturity, and size distribution across the range. Three years of sampling lobsters from Baja California Mexico (to the south) to the Channel Islands (to the north) revealed regional differences in these metrics and that lobsters mature at smaller sizes than have been assumed in management models.
ROCKY REEF ECOLOGY
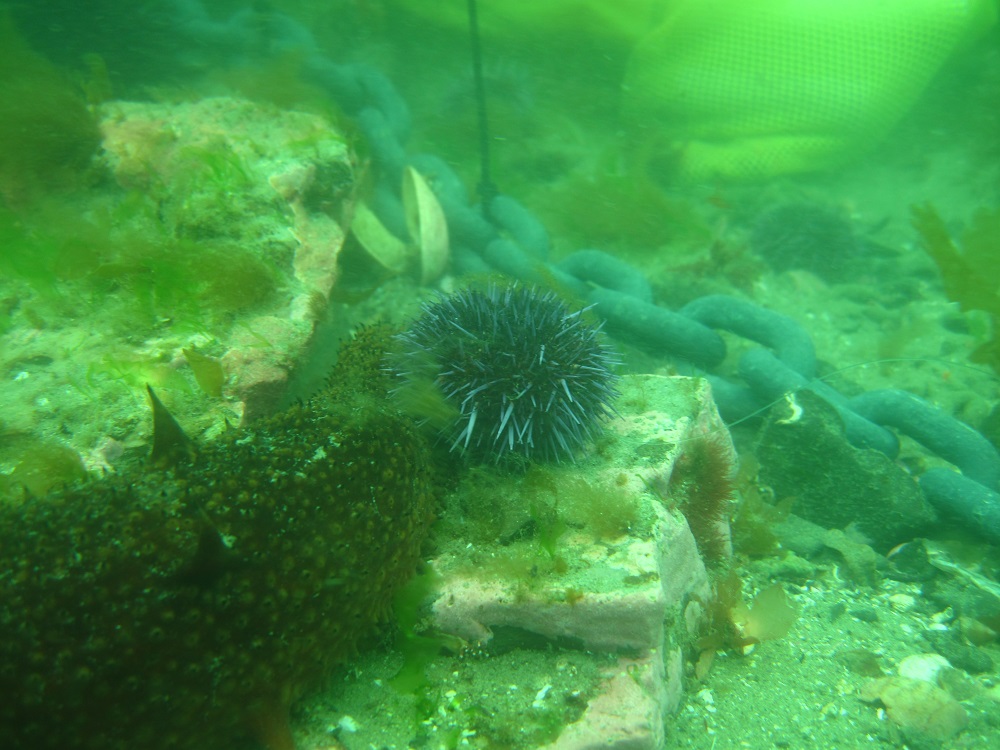
Relevant publications:
*Dunn, R.P., M.L. Baskett, and K.A. Hovel. 2017. Interactive effects of predator and prey harvest on ecological resilience of rocky reefs. Ecological Applications 27: 1718-1730.
*Nichols, K.D., **L. Segui, and K.A. Hovel. 2015. Effects of predators on sea urchin density and habitat use in a southern California kelp forest. Marine Biology 162: 1227-1237.
Former graduate student Julia Coates conducted several experiments with pink abalone on rocky reefs in San Diego. Julia tested how far abalone move within reefs, and whether they form spawning aggregations that enhance reproductive success. Her work had strong implications for strategies that may lead to recovery of these severely depleted populations.
Relevant publications:
*Coates, J.H., K.A. Hovel, J.L. Butler, and A.J. Bohonak. 2014. Recruitment and recovery of pink abalone (Haliotis corrugata) in a historically overexploited kelp forest: are local populations self-sustaining? Journal of Experimental Marine Biology and Ecology 460: 184-192
*Coates, J.H., K.A. Hovel 2014. Incorporating movement and reproductive asynchrony in a simulation model of fertilization success for a marine broadcast spawner. Ecological Modelling 283: 8-18.
*Coates, J.H., K.A. Hovel, J.L. Butler, A.P. Klimley, and S.G. Morgan. 2013. Movement of a broadcast spawning marine invertebrate: implications for restoration strategy and population recovery of pink abalone (Haliotis corrugata). Marine Ecology Progress Series 486: 189-201.